Exploring the lakes of Southern Greenland has been the most professionally joyful time of my career as an evolutionary ecologist. Yearly trips over the past 6 years (with the exception of 2020!) have been a continual source of scientific inspiration. In 2024, the Swiss Polar Institute (SPI) funded an Exploratory Grant that I wrote with Jakob Brodersen (also a Senior Scientist at Eawag) to study The Evolution of Lake Ecosystems and their Biological Communities. Melting glaciers are creating new lakes in the landscape that become home to biologically diverse communities. We are interested in how these communities assemble, how organisms adapt to changing environmental conditions, and how new species evolve. More about that later, after a few comments about motivation, maps, and reindeer dogs.
Why study evolutionary ecology in Greenland?
Fieldwork in remote locations is challenging and slow. It is much easier, safer, and more efficient to study organisms in a laboratory or field setting closer to home. Our scientific institutions and funding bodies increasingly value efficiencies that manifest in more scientific papers and student theses. These are some of the outputs and currencies that professional scientists live by. But science also has a long tradition of exploration and discovery, and of training the next generation to do so. Stephen Carpenter, a well-known ecologist once quipped, “Laboratory experiments keep ecologists on campus, where administrators would like them to be, instead of travelling to remote field sites“ (Carpenter 1996). He argues that some processes can’t be studied in small-scale settings. For example, to understand the ecosystem impacts of climate change, we need diverse teams of researchers off campus, observing and learning how to document ecosystem change.
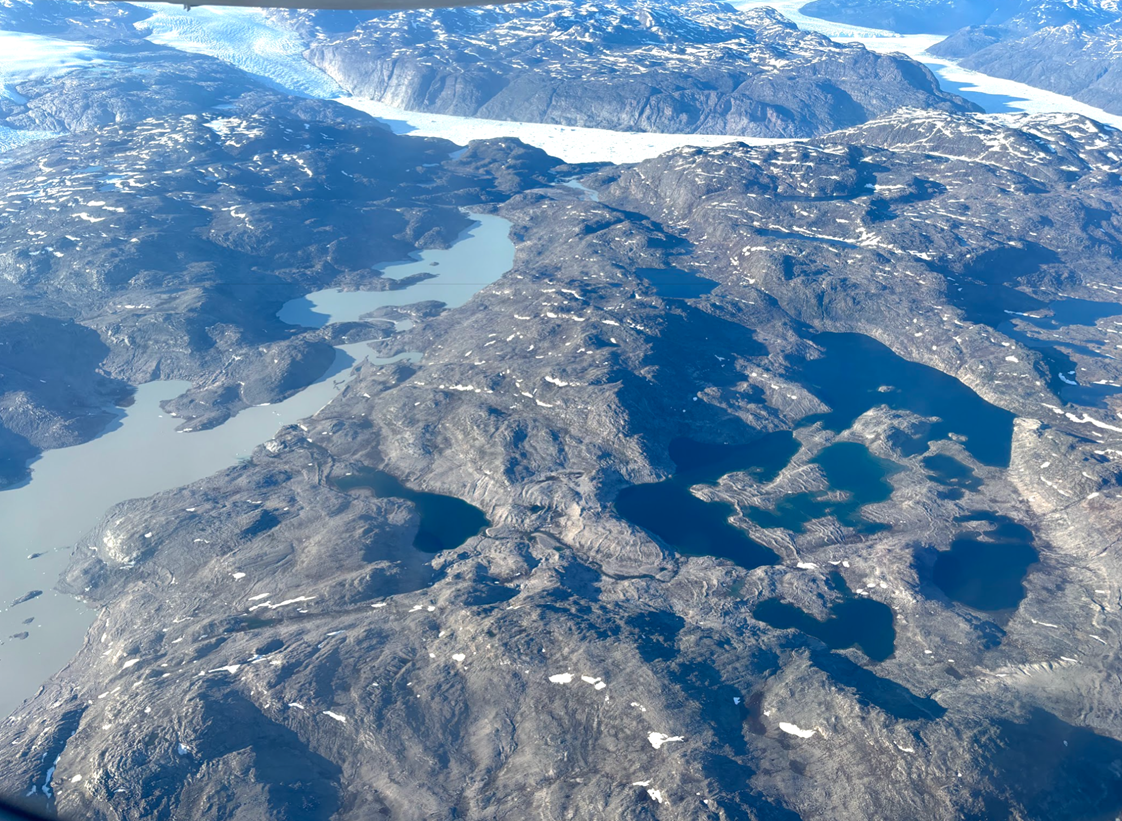
© 2024 Blake Matthews, all rights reserved
Carpenter further argues that there is a cognitive danger that small-scale thinking will canalise a generation of students to become “larval microcosmologists” who study highly abstracted study systems in overly simplified environmental conditions. I am guilty of this, having done many experiments in simplified ecosystems, ranging from tiny vials (5 mL) to “larger” outdoor ponds (15,000L). I appreciate both the value and limitations of these experimental settings. But, if I start confusing these highly abstracted systems with the realities of complex ecosystems, then I risk committing Alfred Whitehead’s fallacy of misplaced concreteness (Whitehead 1967). This occurs, as Whitehead warns, when we mistake the conceptual and physical maps of our science (i.e. the set of simplifications we employ when studying laboratory microcosms) with the actual landscapes and ecosystems of interest (e.g. the watersheds of Southern Greenland).
Throughout my career I have been largely content with doing small-scale experiments and distinctly recall being miffed by Stephen Carpenter’s critique when I first read it. But now his words increasingly resonate: “As ecology becomes more and more a science done indoors by urbanites, there is a significant risk of losing our sense of context”. Such a loss of context can reduce our capacity as scientists to contribute to wise decision making about climate-impacted ecosystems. I now increasingly feel a responsibility to train the next generation of field-based scientists, and what better place to do this than in Southern Greenland.
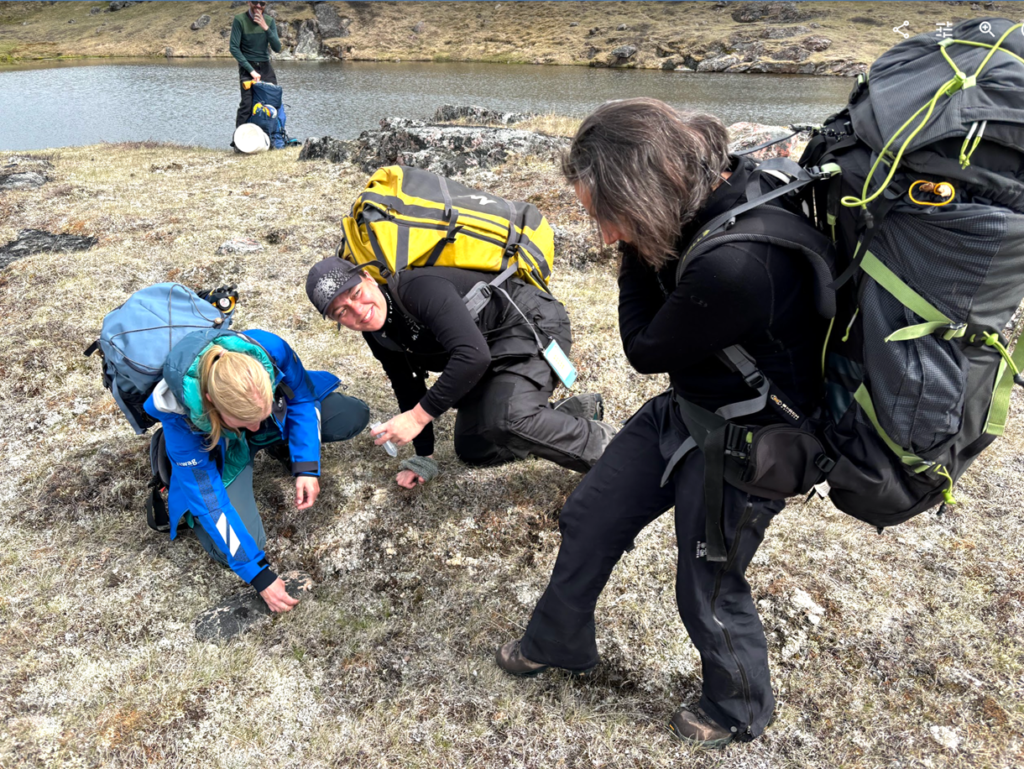
How do we conceptually map the Southern Greenland land and waterscapes?
The scale of Southern Greenland’s fluvial ecosystems are daunting. A massive ice sheet looms over thousands of lakes dotting the landscape – each is home to fascinatingly complex and dynamic ecosystems. Individually these systems are highly worthy of our devoted attention. However, given the enormity of the scales, we need to decide how to spend our limited time, often just a few weeks per year. We need a philosophy for limited beings (Wimsatt and Wimsatt 2007), something to guide our simplifications and steer our effort allocations. Campus learning has barely prepared us for this, and it feels like experiential learning all the way down.
Our first major simplification is to classify lakes based on their food web configuration. Many lakes lack fish, because they don’t have connectivity to the ocean and have limited opportunity for fish colonisation. This is a blessing for us because these fishless lakes are rare in more populated urban settings and, so they serve as useful reference systems for lakes with fish. In Greenland, there are two freshwater fish species, Arctic char (Salvelinus alpinus) and threespine stickleback (Gasterosteus aculeatus), both of which are anadromous fish (i.e. they breed in freshwater but migrate to the ocean as adults). However, both species readily lose their migratory behaviour and establish resident freshwater populations. Stickleback, for example, establish populations and adapt to freshwater lakes and streams – a feat these small fish (adults can reach upwards of 10 cm) have achieved in countless systems in the Northern hemisphere. Arctic char also establish freshwater populations, but these fish reach a much bigger size (i.e. adults are often >50cm), and so they both compete with stickleback for food when they are young, and they can eat stickleback when they are older. Arctic char are also well known for their propensity for forming new species, which happens repeatedly in freshwater lakes. In some of the largest lakes of Southern Greenland, up to seven genetically and ecologically differentiated Arctic char species have been described (Doenz et al. 2019). These adaptive radiations, as they are described by evolutionary biologists, always include a piscivorous species of Arctic char that eats stickleback. A key question in evolutionary biology is how new species are formed, and how species interactions facilitate (or not) the process of speciation. For this reason, understanding the interaction between stickleback and char is a focal point of our research program. It keeps us up at night with endless speculative discussions. It gets us out of bed in the morning to reveal another lake’s insights.
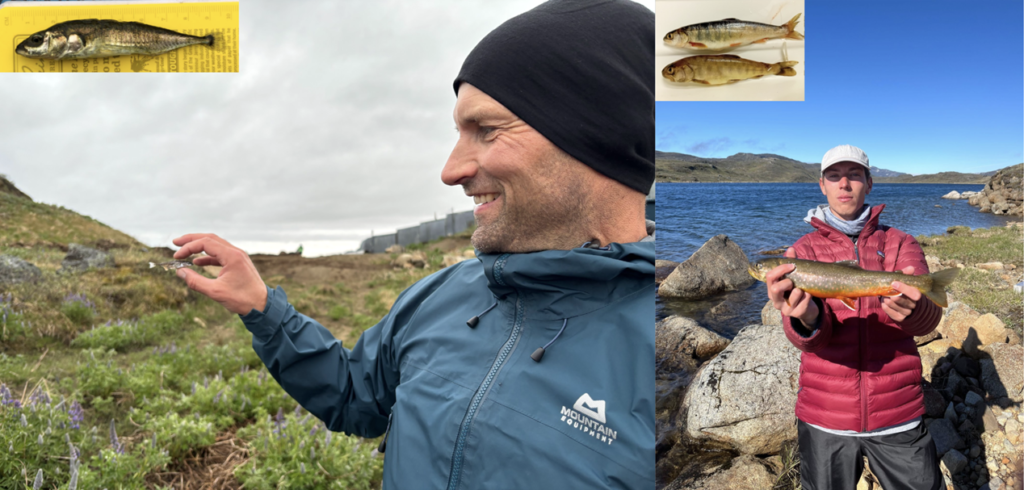
Our second major simplification is to classify lakes based on their position in the landscape and their degree and history of glacial influence. This allows us to reconstruct “in space” the temporal sequence of ecosystem development “over time” – a technique in science called a space-for-time substitution. The vast majority of lakes in the landscape are relatively new, having only emerged since the last retreat of the glaciers about ~15,000 years ago. But, climate change is continually reworking these watersheds. New lakes and river systems are emerging, and lake connectivity to glacial meltwaters is changing. Glacial meltwater is loaded with silt and clay, giving some lakes either a characteristic grey or light blue colour. In these systems light is strongly attenuated, and only reaches the lake bottom close to the shoes, sometimes less than a metre in depth. By comparison, lakes without glacial influence have well-lit bottom waters, and you can see the bottom 10-15 metres below the water surface.
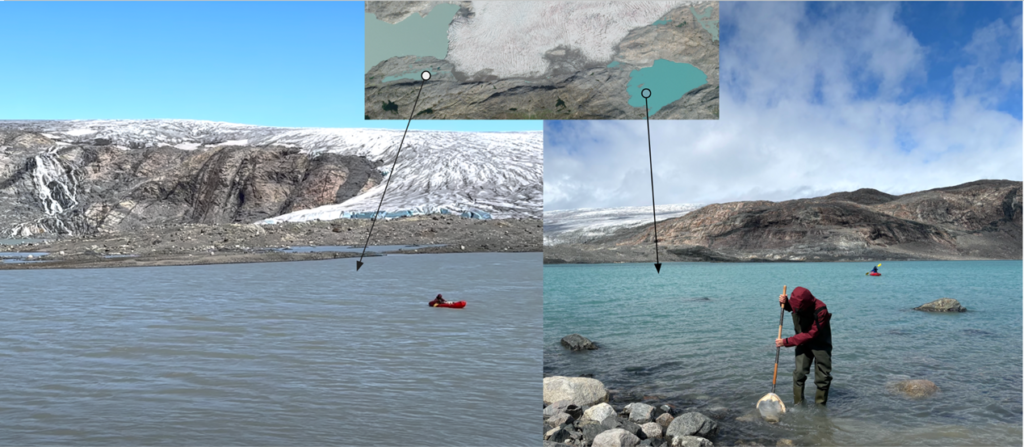
© 2024 Blake Matthews, all rights reserved
When light can reach the lake bottom it allows aquatic plants to take root, and beds of macrophytes that develop become habitats for aquatic invertebrates and foraging and breeding grounds for fish. Poorly lit lakes without macrophytes are relatively devoid of large organisms, and so, we were surprised this year to observe that these highly turbid systems can support resident populations of Arctic char. Both stickleback and Arctic char are readily caught in the adjacent fjords, but only Arctic char seem capable of establishing populations in these turbid systems. In lakes that have recently transitioned from turbid to clear, the Arctic char populations are well established, but stickleback are still absent. Lower in the drainages (closer to the fjords), stickleback populations are well established, have adapted to be resident in lakes, and co-occur with Arctic char.
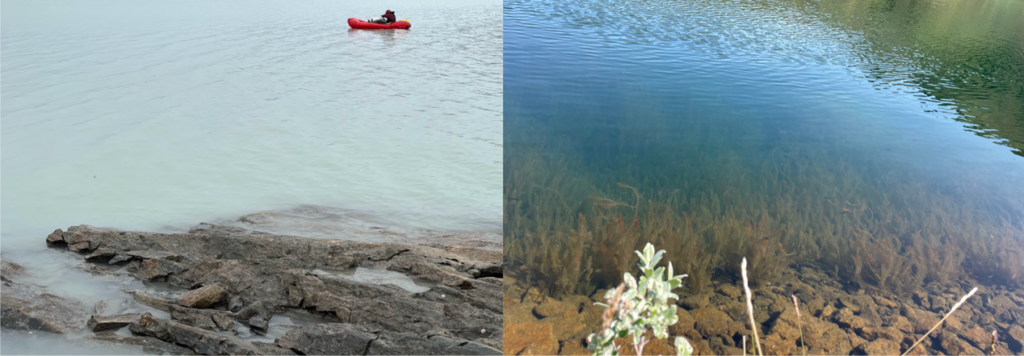
Understanding the colonisation history and arrival order of stickleback and char is another major goal that drives our research interests in this region. In the older lakes it’s hard to uncover the history of food web development and connectivity with glaciers and the ocean. In these systems, for example, neither altitude nor distance to the ocean is a strong predictor of whether a lake has char, stickleback, or both. To help us reconstruct the history of ecosystem development, we needed to study lakes at different stages of ecosystem development, including those having newly emerged in the landscape, recently lost glacial influence, and highly developed food webs. This is where the SPI Exploratory Grant was so helpful, and our starting point for this exploration. This is what brought us to the Isortoq Reindeer Farm.
On reindeer and fish?
Sometimes seeming chance events decades in the past culminate in serendipitous outcomes in the present. In 1990, Stefan Hrafn Magnuson founded a settlement in Isortoq, and, equipped with his Scandinavian knowledge of reindeer herding, he established the first reindeer farm in Southern Greenland. For the ensuing decades he has expanded the family-run farm, and there are now thousands of reindeer roaming the wilderness. You can read about his life story in the book ISORTOQ: Stefan hreindyrabondi, written by Svava Jonsdottir. But good luck finding a copy! You might have to visit the farm to read the book. Isortoq has an incredible history, visited by dignitaries and despots the world over, drawn to the chance of big game hunting. But for us, we were hunting tiny fish and even tinier insect larvae and plankton. Stefan’s choice of location was ideally situated for us to explore an incredible diversity of lakes in a reasonably small geographic area – although nothing is really geographically small in Southern Greenland! Over 12 days, we averaged 15 km of hiking and collected water and invertebrate samples from 20 new lakes, putting us well over 100 lakes for our lifetime Southern Greenland totals. Each lake we sampled was hard fought, either because it was pouring rain, at high elevation, infested with bugs, or excessively windy. But, many were staggeringly beautiful. Even after 5 years of lake sampling in Southern Greenland, this landscape was both emotionally and scientifically inspiring.
Stefan’s deep knowledge of the landscape was a huge asset for us. We started close to the farm, sampling older clearwater lakes that seemed very familiar to us from our time in other lake districts of Southern Greenland, for example near the settlement of Qassiarsuk, and on the islands of Tututtoq and Akia. After a few days we started sampling the more turbid lakes influenced by glaciers – this forced us to adapt to several new challenges. These systems were further away and sometimes at higher elevation, and had so little productivity that we needed to collect double the volume of water to do our analyses. This meant heavier bags carried over longer distances. But, we are a well seasoned team! Myself, Danina Schmidt (a Eawag biologist) and Marek Svitok (a professor from Slovakia) have worked together on two previous expeditions in Southern Greenland, and we were up for the challenge.
One thing you learn quickly when doing fieldwork is that a field team only works as well as it is fed. Most days we ate wraps, with slices of apples, cheese, and dijon mustard. It’s a lightweight and compact lunch, with enough calories to get us through the day – with some additional snacking. We also have some rules that kept us going. Here is a selection from our rather long, and currently unpublished, list of fieldwork wisdoms. Never talk about food you don’t have. Know where all your stuff is all the time. Drink water, even on rainy days. No wearing waders in the boat (it’s a drowning risk!). Swim responsibly around icebergs. Icebergs are ice-cube sources. Sleep enough. Drive slower than the locals. Wear field clothes in the field. Bake occasionally. Dinner is sacred. Sago soup is pretty good. Pack the boat dry or it will rot.
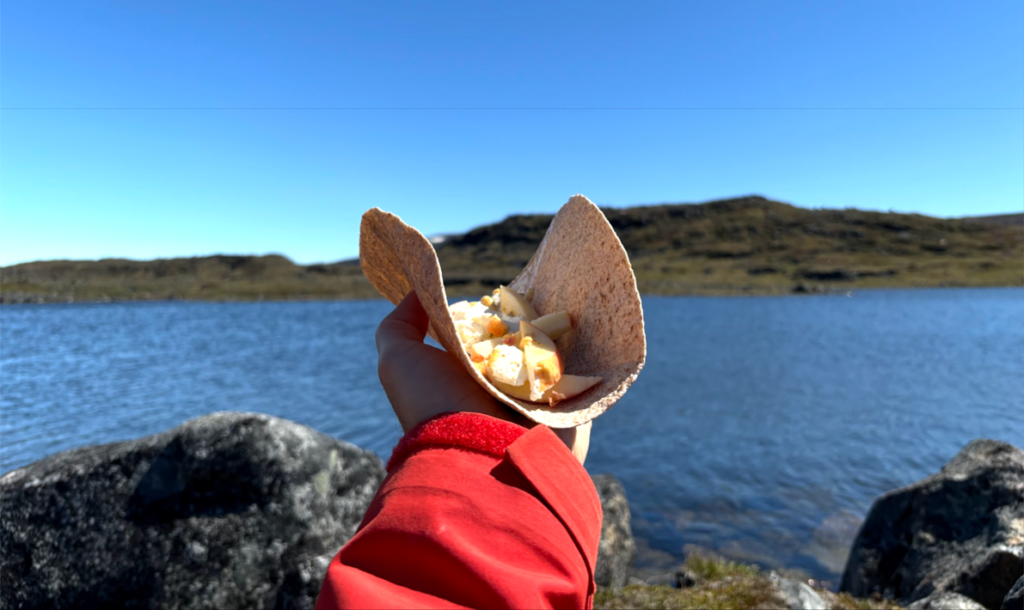
In the last few days of our trip, we found a lake district unlike anything we had ever sampled before. Thirty years ago, Stefan had placed a cairn of stones at the base of the ice sheet. He no longer hikes out to the ice sheet because of a leg injury he sustained in what sounded like a heroic rescue operation decades ago. With some direction from him, we found the cairn, but it was now several kilometres from the current ice sheet edge. In the wake of the retreating ice sheet, a whole network of lakes has emerged in the landscape. A glacial moraine now separates the older from the newer landscape. The older landscape has more soil and plant development, and the newer system is rocky with sandy soils.
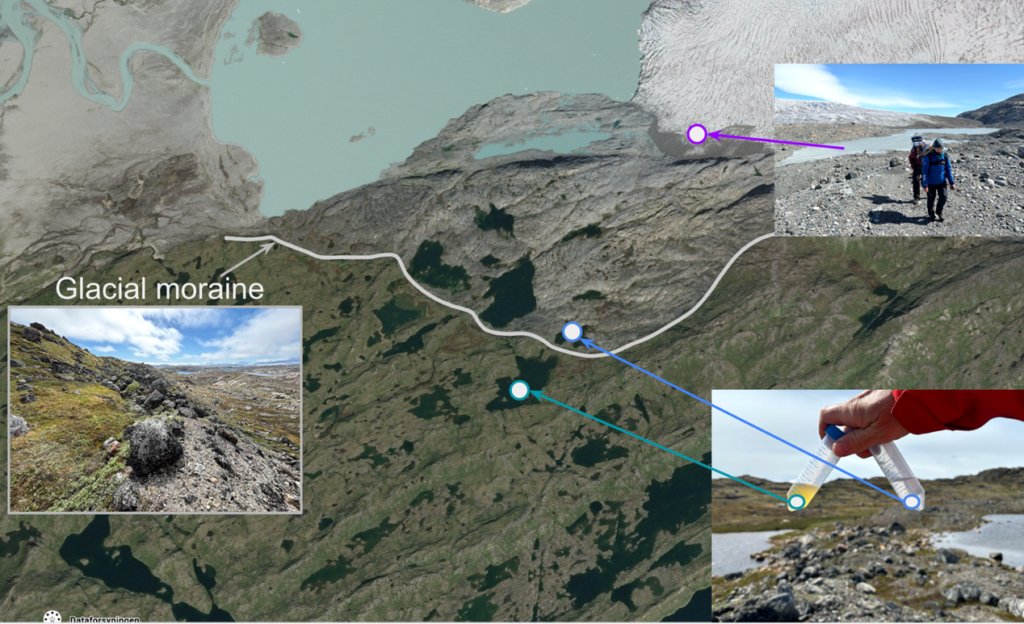
Interestingly, the lakes seem to mirror this pattern. The older lakes have well-developed layers of organic sediment, whereas the newer lakes have largely clay-based sediments. Importantly, this clay can be a hazard for unwary lake samplers. We learned the hard way that this nearshore sediment will hold a boot like hardened cement. We almost lost a pair of waders when the boot got suction-sealed into the clay. I had a knife in my hand ready to cut the boot loose just before it finally popped out.
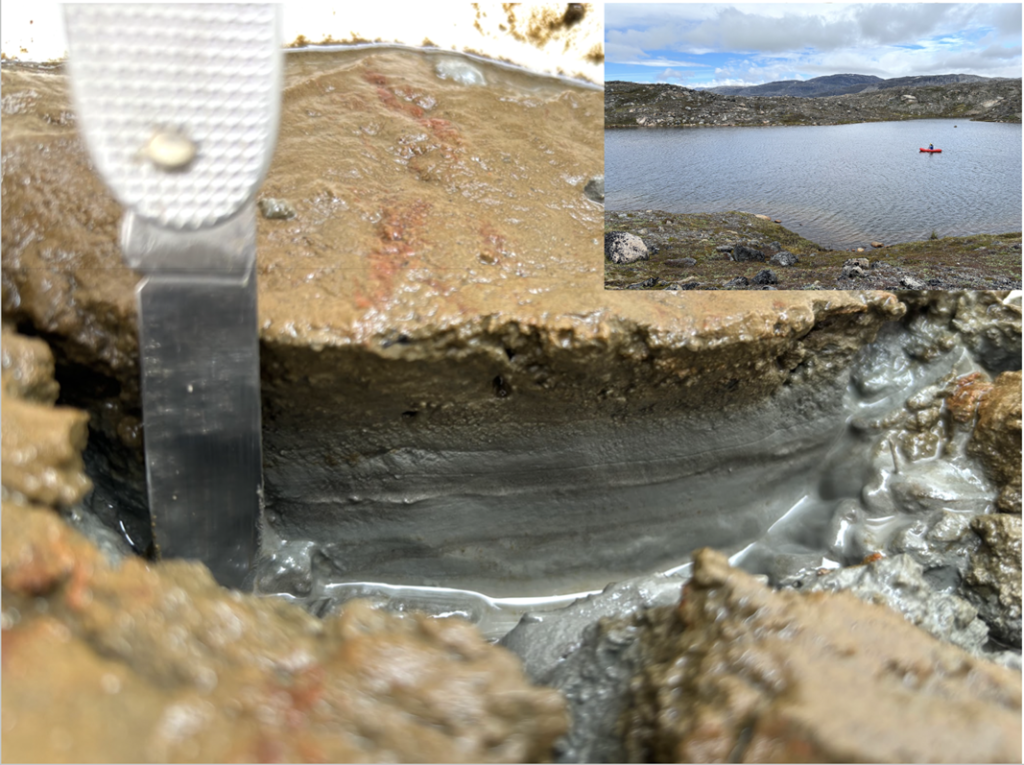
Apart from the lakes, the other novelty of sampling this landscape was of course the reindeer. They were fun. They pop over the ridge, inspect us, and then take off. They also leave their antlers behind.
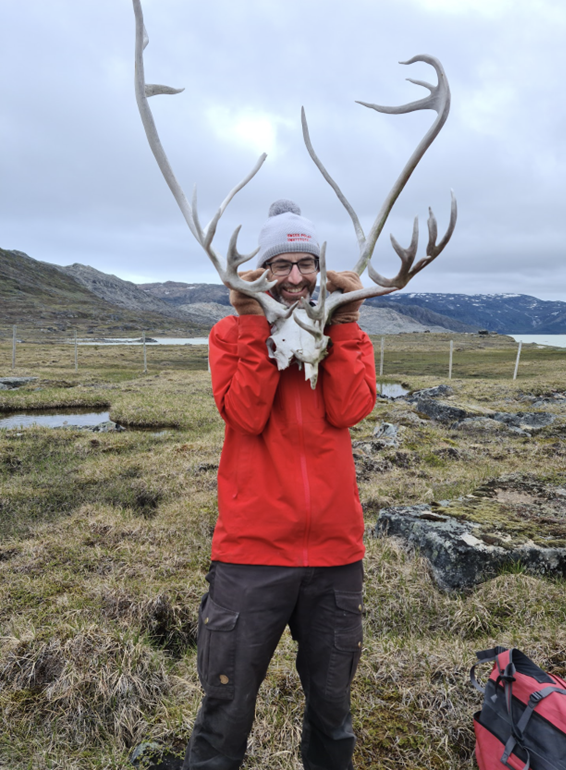
Perhaps more fun than the reindeer was the dog at the reindeer farm – a beautiful Icelandic shepherd. He joined us on one of our 20-km field days to the ice sheet. Unbeknownst to us, he wasn’t supposed to leave the farm. But he knew it! While on our trek together he occasionally chased reindeer to us and also managed to scare many others away. It wasn’t until our return to the farm that we learned that he was still in training as a reindeer herder and wasn’t yet allowed to join the professional herding trips. But he had a good day of fieldwork with us and seemed perfectly trained for a day of professional lake sampling.
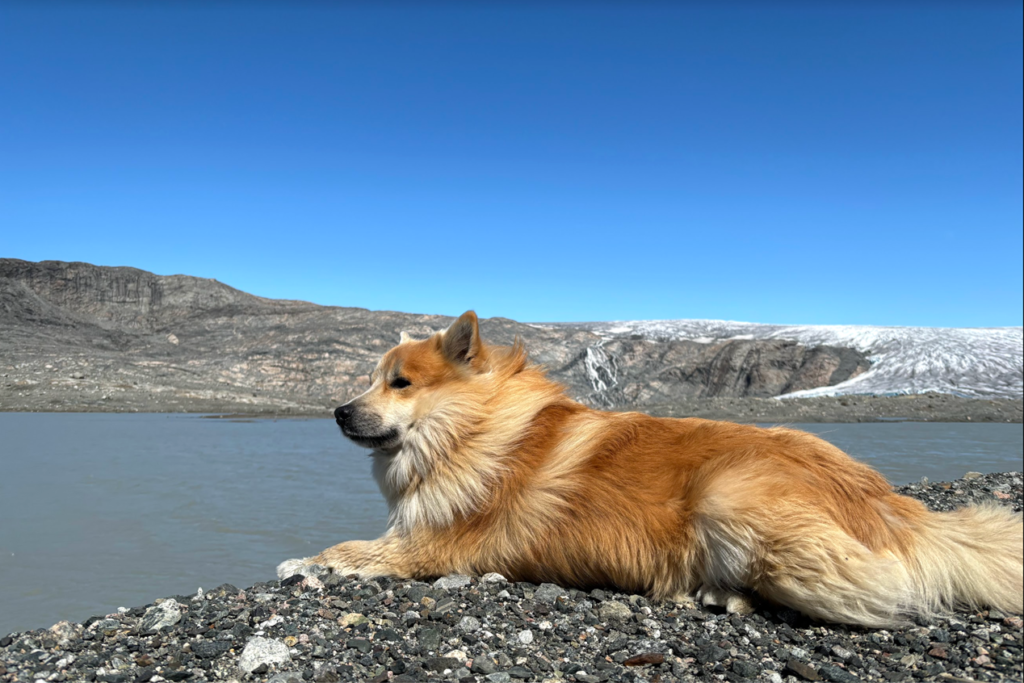
Another major motivation for our work in Southern Greenland is to understand not only how organisms adapt to changing environments, but also how they shape the development of ecosystems. For example, as systems become disconnected from glacial influence what path of ecosystem development will they take? Central to this is not just knowing which species are where and when they arrive, but also how the development of ecosystems is shaped by the organisms themselves. Organisms not only passively adapt to ecosystems that are shaped by external forces, such as meltwater, sunlight, and temperature. As organisms colonise lakes they can also affect the nutrient balance, the water transparency, and establishment of new species. For example, as macrophytes develop, we expect invertebrate communities to flourish and set the stage for subsequent establishment of fish populations. Macrophytes engineer the ecosystems, by affecting nutrient and organic matter development in the sediments and light transparency in the water column. Once fish arrive, things get even more interesting, or at least interesting in a different way. We know from previous experiments that stickleback can dramatically alter the species composition and size structure of prey communities, and this has cascading impacts on the lower trophic levels, leading to an increase in the biomass production of plants. However, what we still want to learn is how stickleback might potentiate the evolution of new Arctic char species, giving them the opportunity to fill a predatory niche afforded by the presence of stickleback. At the moment, this is just a nightcap story that will likely take us a decade or more to resolve. But, what better way to spend time off campus and in the wilds of Southern Greenland!
Securing our future
We live in the Anthropocene, an epoch in which we fundamentally are transforming the ecosystems that sustain life on this planet. Human activities change how species evolve in these ecosystems, and by doing so, how organism-ecosystem interactions develop in the future. We can never decouple our survival on this planet from the ecosystems that sustain us. At best, we can hope that our ecosystem impacts feedback only weakly to affect the survival of future generations. But to prepare for the worst, we need to train the next generation of ecosystem ecologists and cultivate their contributions to society – a society that is in desperate need of a new ecosystem ethic. We thank the Swiss Polar Institute for their continued support of early-career researchers and fieldwork at the frontiers of climate change research.
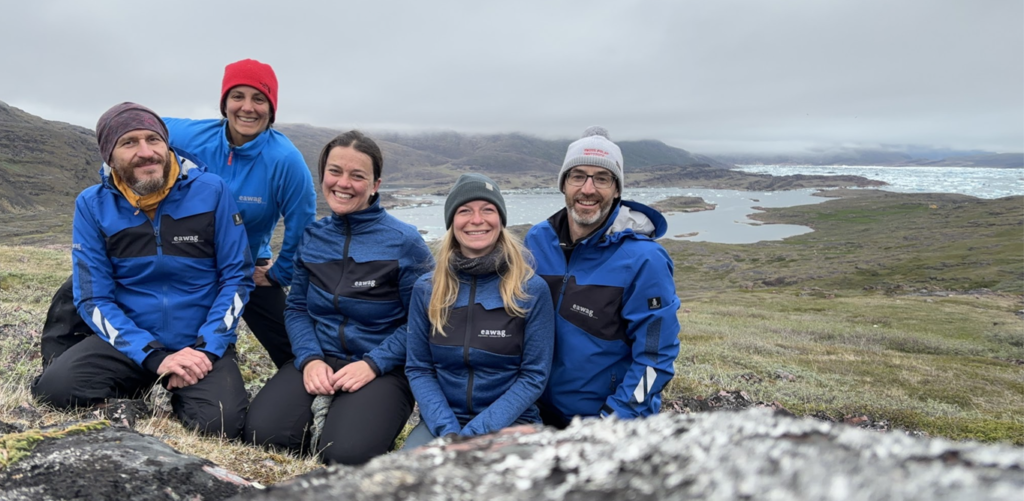
Cited work:
Carpenter, Stephen R. 1996. “Microcosm Experiments Have Limited Relevance for Community and Ecosystem Ecology.” Ecology 77 (3): 677–80.
Doenz, Carmela J., Andrin K. Krähenbühl, Jonas Walker, Ole Seehausen, and Jakob Brodersen. 2019. “Ecological Opportunity Shapes a Large Arctic Charr Species Radiation.” Proceedings. Biological Sciences / The Royal Society 286 (1913): 20191992.
Whitehead, Alfred North. 1967. Science and the Modern World. London, England: Simon and Schuster.
Wimsatt, William C., and William Kurtz Wimsatt. 2007. Re-Engineering Philosophy for Limited Beings: Piecewise Approximations to Reality. Harvard University Press.
Blake Matthews is Research Group Leader in Eco-Evolutionary Dynamics at Eawag in Kastanienbaum, Switzerland. His fieldtrip took place in summer 2024 with financial support from an SPI Exploratory Grant.